|
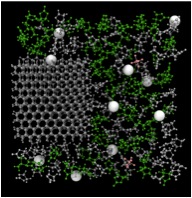
|
Electrochemisty of Li ion batteries
Your battery is low, time to charge it. What happens at the atomic level
when you connect your battery to the charger? Is there a way to charge your
battery faster? These are the types of questions that this research seeks
to answer. The figure is the cover art of a 2010 publication. It shows a
snapshot of a half-cell of a lithium ion battery. The spheres are the lithium
ions, the hexagonal structure is an electrode composed of graphitic sheets,
and the white, red, and green molecules are the electrolyte and counter ions.
The molecular dynamics simulation addresses the question of how long it takes
under various conditions for a lithium ion to intercalate between the graphitic
sheets.
[MAN192 2011] [MAN186 2010] [MAN182 2009] [MAN177 2008] [MAN102 1999]
[MAN101 1999] [MAN090 1997]
|
|
Dynamics of quantum spins interacting with quantum bath
If you want to design and build a quantum computer you should first simulate
a quantum subsystem interacting with a quantum bath to understand the
decoherence and thermalization processes. Such simulatations of the
time-dependent Schrödinger equation (in real time) has been performed
for different spin subsystems interacting with various types of quantum baths.
For particular types of interactions between the subsystem and the bath a
novel algorithm that allows for large baths has been devised.
The top figure is the lattice studied, with the four spins of
the subsystem in cyan and the bath spins in red. The bottom
figure illustrates how adding additional randomness decreases
the time for a measure of decoherence to decrease to the
theoretical value (black horizontal line).
[MAN197 2012] [MAN195 2012] [MAN194 2012] [MAN189 2011]
|
|
Spanning disparte time scales:
Projective Dynamics (PD) and
Monte Carlo with Absorbing Markov Chains (MCAMC)
How do you span disparate time scales that are relevant for materials simulations?
For example, magnetic nanoparticles on the atomic level have time scales related to
an inverse phonon frequency (about 10-13 seconds), while such
nanoparticles are used to not only store your computer data (many years, say
106 sec) but to measure reversals of the Earth's magnetic field
(1013 sec). Novotny and collaborators and students have devised and
implemented two algorithms that can span such disparate time scales: the
MCAMC and PD algorithms. These two algorithms work for all simulations,
including those without a free energy.
[MAN190 2011] [MAN187 2010] [MAN185 2010] [MAN183 2009] [MAN168 2006]
[MAN166 2006] [MAN159 2004] [MAN153 2004] [MAN151 2004] [MAN143 2003]
[MAN142 2003] [MAN141 2003] [MAN137 2002] [MAN130 2002] [MAN127 2002]
[MAN121 2001] [MAN116 2000] [MAN112 2000] [MAN105 1999] [MAN103 1999]
[MAN097 1998] [MAN095 1998] [MAN093 1998] [MAN091 1998] [MAN089 1997]
[MAN075 1995] [MAN072 1995]
|
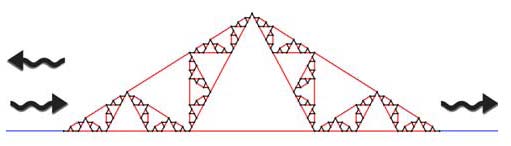
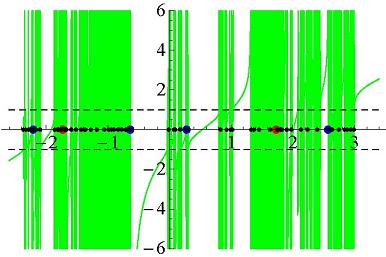
|
Quantum Transport
In high school physics you learn about Ohm's law for electrical resistance.
However, this emperical 'law' comes ultimately in certain limits
from the quantum behavior of electrons in materials. Therefore, the calculation
and understanding of quantum scattering behavior of electrons is of paramount
importance. We have devised a renormalization group (RG) method of calculating
such quantum scattering the in tight-binding approximation. The top figure shows
a quantum wave/particle impinging from the left, being partially reflected to the
left and partially transmitted to the right. The model for the tight-binding
material here is a special type of network, called the Hanoi network where every
node has three connections. The bottom figure shows the mathematical flows
(vertical axis) of the
RG for this Hanoi network. There are interspersed regions as a function of the
energy of the incoming wave/particle (horizontal axis) where the transmission
probability is either very high or very low (related to a small or large
electrical resistance).
[MAN193 2012] [MAN191 2011] [MAN188 2010] [MAN176 2007]
|
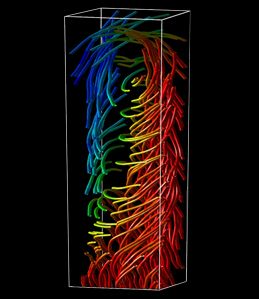
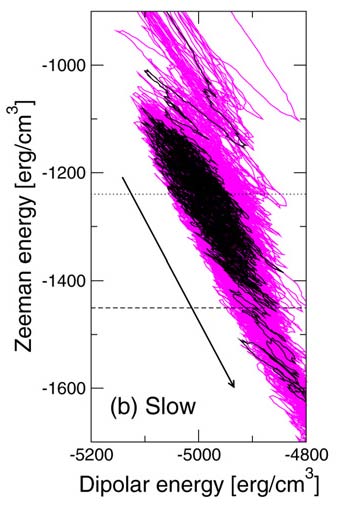
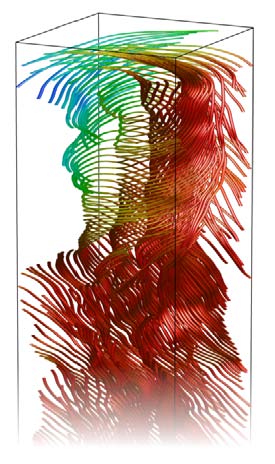
|
Magnetic Nanopillars:
Magnetic nanoparticles are used in a variety of
engineering applications --- where do you think your data is stored on your
hard drive? Therefore, understanding the dynamics of the magnetic behavior
of magnetic nanopillars is important both for purely scientific pursuits
and for engineering design. The two pictures show the field lines of the
magnetization of a nanoscale pillar (top) and of just the endcap portion of
the pillar (bottom). The center figure shows two contributions to
the energy of a pillar for different switching behaviors (black is a slow
switching event while violet is a fast switching event).
[MAN185 2010] [MAN181 2009] [MAN173 2007] [MAN157 2004] [MAN156 2004]
[MAN152 2004] [MAN143 2003] [MAN139 2003] [MAN129 2002] [MAN124 2001]
[MAN123 2001] [MAN120 2001] [MAN115 2000] [MAN107 1999] [MAN104 1999]
[MAN102 1999]
|
|
|
|